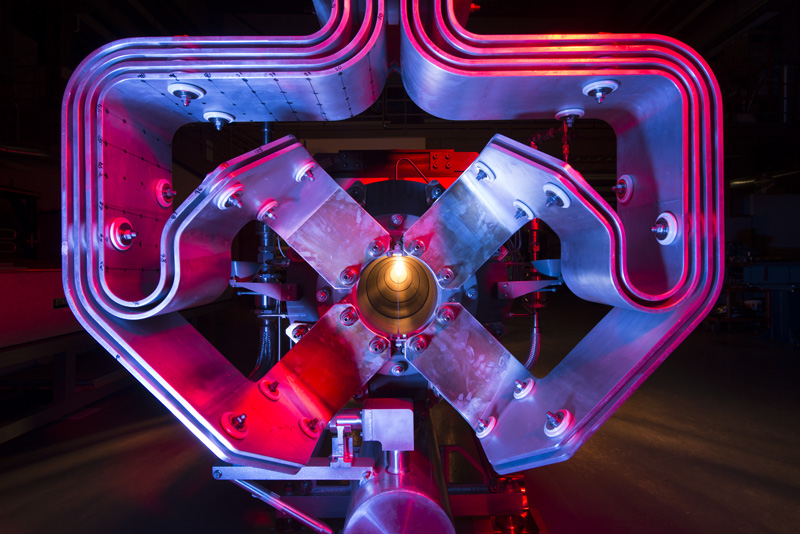
‘Ghostly’ neutrinos provide new path to study protons
Scientists have discovered a new way to investigate the structure of protons using neutrinos, known as ‘ghost particles’
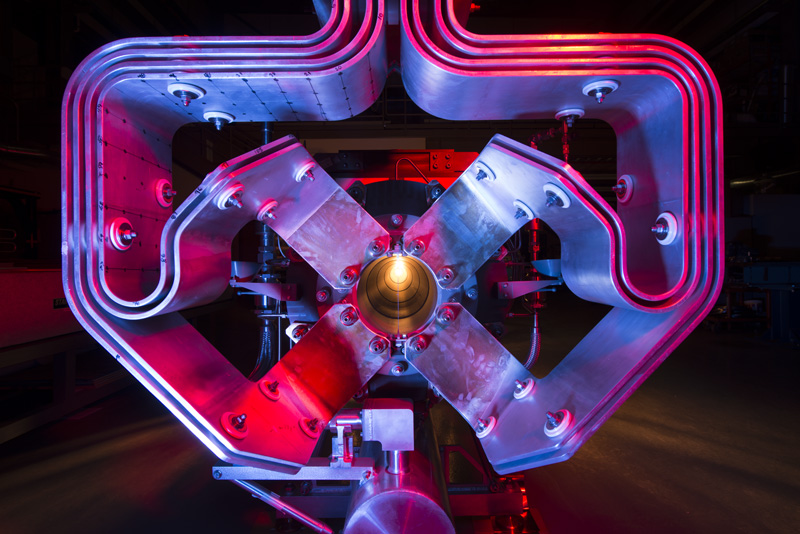
TORONTO, Feb. 1, 2023 – Scientists are that much closer to understanding protons today after using a novel technique involving a high-energy neutrino beam to precisely measure their size, which could change how these kinds of experiments are done and answer many more questions, say researchers from York University.
“We need detailed information about protons to answer questions like which neutrinos have more mass than others and whether or not there are differences between neutrinos and their anti-matter partners,” says Tejin Cai, York University postdoctoral researcher and lead author of the paper published today in Nature. “Our work is one step forward in answering the fundamental questions about neutrino physics that are the goal of these big science projects in the near future.”
The research involved a series of experiments with neutrinos, often referred to as “ghost particles,” over nearly a decade. It was part of the international MINERvA collaboration, which studies neutrinos at Fermi National Accelerator Laboratory (Fermilab).
“While we were studying neutrinos as part of the MINERvA experiment, I realized a technique I was using might be applied to investigate protons,” says Cai, who did the research, involving an international team of scientists, while completing his PhD in the lab of Kevin McFarland, the Dr. Steven Chu Professor in Physics and the Acting Vice Provost for Academic Affairs at the University of Rochester.
They found that the proton radius as seen by neutrinos is 0.73 femtometres – a quadrillionth of one metre.
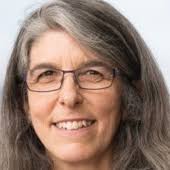
“When we proposed MINERvA, we never thought we’d be able to extract measurements from the hydrogen in the detector,” says Professor Deborah Harris, a particle physicist in York’s Faculty of Science, a senior scientist at Fermilab and a co-spokesperson at MINERvA. “Making this work required great performance from the detector, creative analysis from scientists, and years of running the most intense high-energy neutrino beam on the planet.”
That’s the novel part of this experiment. The use of a beam of neutrinos to investigate the structure of protons was once thought impossible. The MINERvA group used a high-power, high-energy particle accelerator, which produces the strongest source of high-energy neutrinos on the planet. This new technique offers scientists a new way of looking at the small components of an atom’s nucleus.
Although neutrinos are one of the most abundant particles in the universe, they are notoriously difficult to detect and study as they don’t have an electrical charge and nearly zero mass. They are often referred to as “ghost particles” because they rarely interact with atoms, but they play a large role helping scientists answer fundamental questions about the universe.
Atoms, and the protons and neutrons that make up an atom’s nucleus, are so small that researchers have a difficult time measuring them directly. Instead, they build a picture of the shape and structure of an atom’s components by bombarding atoms with a beam of high-energy particles. They then measure how far and at what angles the particles bounce off the atom’s components.
For example, if marbles were thrown at a box, they would bounce off it at certain angles, enabling someone to determine where the box was, its size and shape – even if the box was not visible.
“This is a very indirect way of measuring something, but it allows us to relate the structure of an object – in this case, a proton – to how many deflections we see in different angles,” says McFarland.
A new technique
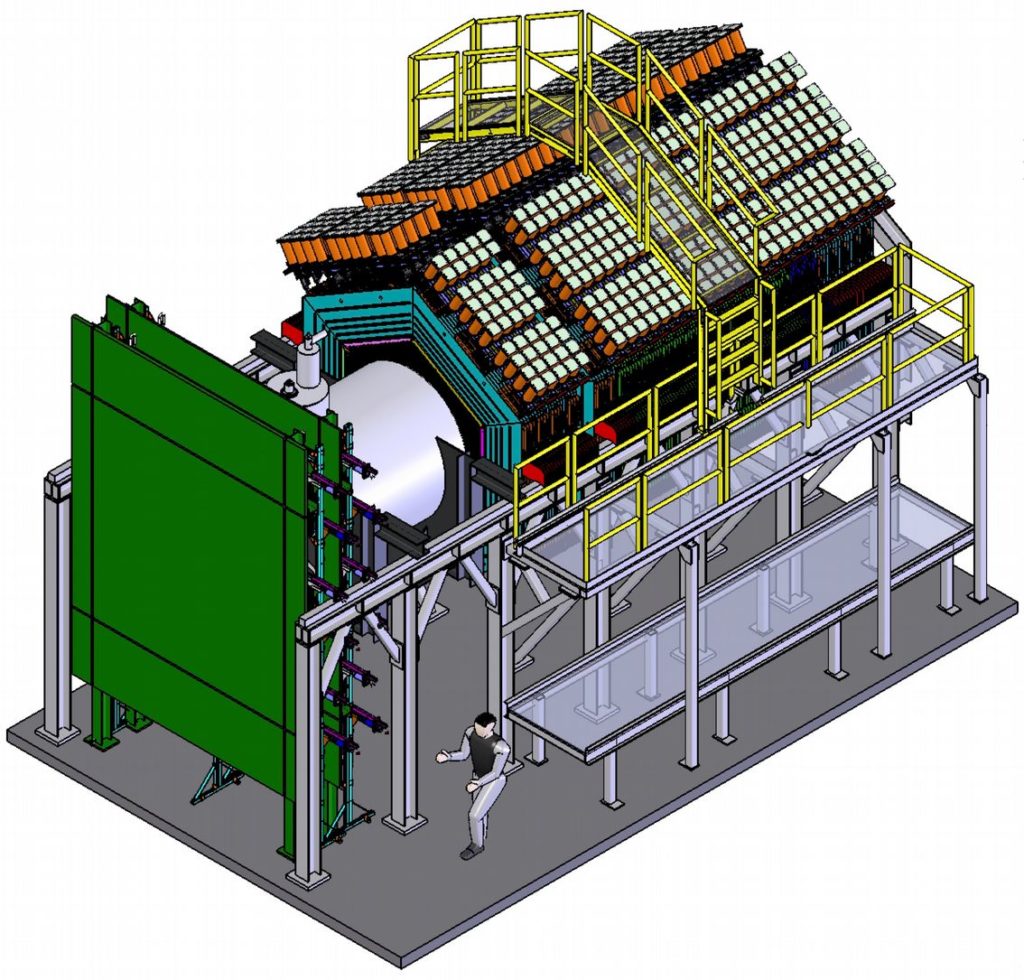
Specifically, the researchers are hoping to use the technique to separate the effects related to neutrino scattering on protons from the effects related to neutrino scattering on atomic nuclei, which are bound collections of protons and neutrons.
“Our previous methods for predicting neutrino scattering from protons all used theoretical calculations, but this result directly measures that scattering,” says Cai.
McFarland adds, “By using our new measurement to improve our understanding of these nuclear effects, we will better be able to carry out future measurements of neutrino properties.”
What is a neutrino?
Neutrinos are created when atomic nuclei either come together or break apart. The sun is a large source of neutrinos, which are a byproduct of the sun’s nuclear fusion. If you stand in the sunlight, for example, trillions of neutrinos will harmlessly pass through your body every second.
Even though neutrinos are more abundant in the universe than electrons, it is harder for scientists to experimentally harness them in large numbers; neutrinos pass through matter like ghosts, while electrons interact with matter far more frequently.
“Over the course of a year, on average, there would only be interactions between one or two neutrinos out of the trillions that go through your body every second,” says Cai of York’s Faculty of Science. “There’s a huge technical challenge in our experiments in that we have to get enough protons to look at, and we have to figure out how to get enough neutrinos through that big assembly of protons.”
A chemical trick
The researchers solved this problem in part by using a detector containing a target of both hydrogen and carbon atoms. A target of pure hydrogen wouldn’t be sufficiently dense for enough neutrinos to interact with the atoms.
“We’re performing a ‘chemical trick’, so to speak, by binding the hydrogen up into hydrocarbon molecules that make it able to detect sub-atomic particles,” McFarland says.
To isolate only the information from the hydrogen atoms, the researchers then had to subtract the background “noise” from the carbon atoms.
“The hydrogen and carbon are chemically bonded together, so the detector sees interactions on both at once,” Cai says. “I realized that a technique I was using to study interactions on carbon could also be used to see hydrogen all by itself once you subtract the carbon interactions. A big part of our job was subtracting the very large background from neutrinos scattering on the protons in the carbon nucleus.”
The collective expertise of MINERvA’s scientists and the collaboration within the group was essential in accomplishing the research, says Cai.
“The result of the analysis and the new techniques developed highlight the importance of being creative and collaborative in understanding data. While a lot of the components for the analysis already exist, putting them together in the right way really made a difference, and this cannot be done without experts with different technical backgrounds sharing their knowledge to make the experiment a success.”
The paper, Measurement of the axial vector form factor from antineutrino-proton scattering, is published today in the journal Nature.